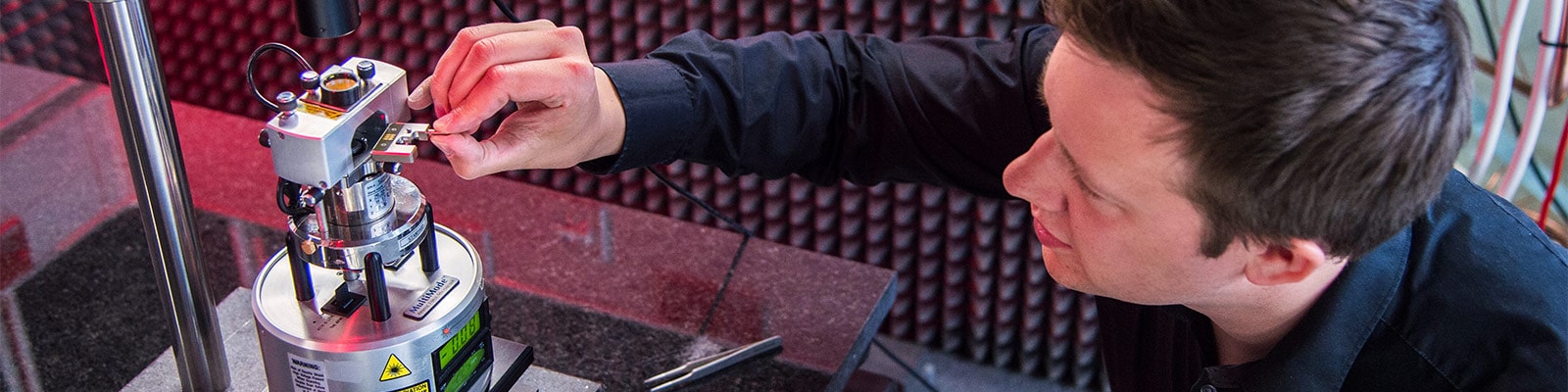
About us
We are a collaborative community of researchers interested in the development, study and exploitation of quantum phenomena, nanostructured materials and nanoscale devices.
accordion
We study quantum devices through low-temperature electronic transport measurements. Our goal is control at the level of single charge, single flux quantum, single photon and single phonon, enabled by fundamental physical phenomena such as superconductivity, the Josephson effect, flux and charge quantisation, and quantum entanglement.
The ability to control and measure quantum states in nanoscale solid-state devices makes them a promising platform for new quantum technologies. Applications include quantum computing and quantum simulation, quantum encryption, quantum metrology, and novel sensors operating beyond the standard quantum limit.
Work within this activity includes:
- Quantum Metrology
Superconducting and hybrid charge pumps for accurate definitions of the ampere. - Graphene & 2D Materials
Transport phenomena in 2D materials, including hydrodynamic flow and the superconducting proximity effect. - Ultralow temperature thermometry and devices
Developing the techniques required to undertake quantum transport measurements at electron temperatures below one millikelvin. - Semiconductors
Transport properties of narrow band-gap semiconductor heterostructures and nanostructures. - Nanoelectromechanics
Using nanoscale cantilevers to probe properties of quantum fluids.
Team
- Professor Yuri Pashkin
- Professor Rich Haley
- Professor Manus Hayne
- Dr Sergey Kafanov
- Dr Leonid Ponomarenko
- Dr Jon Prance
- Dr Viktor Tsepelin
Research involves the design, fabrication and characterisation of novel quantum devices that exploit the properties of III-V compound semiconductor nanostructures such as quantum dots, nanowires, quantum wells and superlattices.
A key aspect of our research is the use of advanced epitaxial growth, including:
- narrow band-gap semiconductors, such as GaSb, InAs and InSb
- self-assembled and site-controlled nanostructures
- droplet epitaxy
- hydrogenation of semiconductors
- dilute nitrides
- mismatched materials with atomically abrupt interfaces on GaAs and silicon substrates
We are developing a variety of photonic and electronic devices, including:
- mid-infrared LEDs and lasers for environmental gas sensing
- solar cells and thermo-photovoltaic devices for renewable energy generation
- GaSb quantum ring lasers for telecommunications
- low-noise photodetectors for focal plane arrays and thermal imaging
- low-voltage non-volatile memories
Our research is frequently carried out in collaboration with industry partners, including IQE, CST, Amethyst, SELEX, Huawei and others, as well as many academic groups worldwide.
Team
- Professor Tony Krier
- Professor Manus Hayne
- Professor Robert Young
- Dr Andrew Marshall
- Dr Qian Zhuang
We explore physical phenomena in advanced materials at the micron, nano and atomic scale. Our activities include:
- the development of functional 'smart' surfaces realised via novel molecular self-assembly methods
- nanoscale device characterisation
- atomic resolution imaging
- instrument development and biomaterial analysis
We develop new materials with targeted chemical, electrical, thermal and catalytic properties such as self-assembled molecular networks and novel 2D material - organic film heterostructures fabricated using a wide range of atomically precise molecular assembly techniques and spanning environments from highly controlled ultra-high vacuum conditions to liquid-phase molecular self-assembly and Langmuir-Blodgett deposition.
Underpinning this research are a wide range of characterisation methods and a long-standing interest in new instrument development. Scanning probe microscopy (SPM) is unparalleled in its ability to provide spatial resolution at the atomic and nanoscale. Our suite of SPM instruments is dedicated to high-resolution imaging, nanomechanical mapping, electrical measurement and scanning thermal microscopy, which we combine with optical spectroscopy, high-speed interferometry, x-ray photoelectron spectroscopy, synchrotron radiation studies and electronic structure calculation providing access to the full spectrum of materials properties.
Team
- Professor Oleg Kolosov
- Dr Sam Jarvis
- Professor Benjamin Robinson
Our research straddles three main disciplines: material science, quantum optics and information security. Through the hybridisation of these fields, we are driving a unique research activity; investigating the application of light-matter interfaces in low-dimensional structures for physical security applications.
We divide our research into the following themes:
- Developing components for optical quantum information processing (QIP) using low-dimensional semiconductor structures. The scalability and low-cost of semiconductor systems make them ideal for producing scalable quantum technologies.
- Harnessing the unique properties of nanostructures embedded in devices for identity provision and authentication. At the atomic level, no two objects are identical. We're developing technologies that produce east-to-read signatures based on this uniqueness, for security applications.
- Quantum light emission from heterostructures of two-dimensional materials, and associated applications. Solid-state lighting and two-dimensional materials were both the subject of recent Nobel prizes in physics. The combination of the two is driving an exciting research field.
Team
- Professor Robert Young
- Professor Manus Hayne
- Professor Benjamin Robinson
-
Group Members
Loading People
Current PhD Opportunities
Quantum Nanotechnology PhDs accordion accordion
Supervisor
Dr Qiandong Zhuang
Description
This project aims to establish new research in III-V nanowires on silicon for advanced photoceptors and/or nanolasers based on silicon
One-dimensional semiconductor nanowires have a number of photonic favourable advantages, such as enhanced light absorption, efficient carrier separation, freedom on device design and non-restrict on substrate. These enable it to be a promising build block for next generation photonic devices such as photodetectors, nanolasers, high-speed transistors and spintronics etc. Due to the readily growth ion silicon substrates, this material also provides a promising route towards integration with silicon circuits. Tis project will develop molecular beam epitaxy of III-V nanowires on silicon or other 2-D materials and to explore its device applications for either photodetectors or lasers.
The student will gain experience and expertise in molecular beam epitaxy, semiconductor materials characterization, device fabrication in cleanroom, and device characterizations (either photodetectors or lasers).
Supervisor
Professor Manus Hayne
Project
Computers are based on the von Neumann architecture in which the processing and memory unit are largely separated, requiring information to be shuffled to and fro, which is inefficient and creates a bottleneck. This is particularly disadvantageous for activities that are memory intensive, such as artificial intelligence and machine learning.
An alternative is in-memory computing [1, 2], in which certain algorithms are performed within the memory unit. This is less flexible than the traditional von Neumann approach, but has huge potential in terms of computational time and energy saved for memory intensive tasks involving operations that are performed huge numbers of times. These could be common logical functions such as AND and OR, or matrix-vector multiplications which comprise between 70% and 90 % of the deep-learning operations in speech, language and vision recognition [2]. Many conventional and emerging memory technologies have been investigated for in-memory computing, such as SRAM, DRAM, flash, phase change memory, resistive RAM, and very recently MRAM [3]. However, memory technologies with very fast, low-energy switching, high endurance (and low disturb) are required to fulfil the potential of in-memory computing and compete with the conventional CMOS-based approach [1].
ULTRARAM™ is a patented Lancaster memory technology with a non-volatile storage time of at least 1000 years, an endurance in excess of 10 million program/erase cycles, non-destructive read, low disturb, a switching energy that is 100 times lower per unit area than DRAM, and intrinsic sub-ns switching speeds [4]. It has huge potential as a conventional memory, but also for in-memory computing.
The PhD project will be the first to investigate ULTRARAM™ for in-memory computing. The research will involve modelling (at different scales), and designing, fabricating and testing some simple ULTRARAM™ for in-memory computing circuits to show proof of principle.
This PhD is offered in collaboration with Quinas Technology. Funding for UK students is available on a competitive basis.
[1] ‘Memory devices and applications for in-memory computing’, A. Sebastian et al., Nature Nanotechnology, 15, 529 (2020) [Link]
[2] ‘In-memory Computing for AI Applications’, E. Eleftheriou, 16th International Conference on High-Performance and Embedded Architectures and Compilers, 18-20 January 2021 [YouTube]
[4] ‘A crossbar array of magnetoresistive memory devices for in-memory computing’, Jung et al., Nature 601, 211 (2022) [Link]
[3] ‘ULTRARAM: a low-energy, high-endurance, compound-semiconductor memory on silicon’, P. D. Hodgson, D. Lane et al. [Link]
Supervisor
Dr Qiandong Zhuang
Description
This project aims to develop advanced sensing technologies based on laser spectroscopy for non-invasive biomedical diagnosis.
There is increasing demand on non-invasive wearable medical sensors capable of providing fast and continuous real-time monitoring of physiological variables, e.g. partial O2 (pO2), partial CO2 (pCO2), glucose, lactate etc, which have close correlation with many chronic diseases and stroke, hence, such medical sensors are pivotal for the management of numerous medical conditions. A typical example is diabetes, 4 million people in the UK have been diagnosed with diabetes[1] — about 10% with type 1, and the rest with type 2, which estimated to be increased to 5 million in 2025[2]. Worldwide, about 415 million adults are living with diabetes, according to the International Diabetes Federation. People with type 1 diabetes may be advised to test their blood-sugar levels four to eight times a day, using invasive needle-prick methods, in order to stay alive. A convenient and non-invasive monitor is a long-standing requirement for management of the condition and would revolutionise patient self-care.
Laser-based spectroscopy has been proposed as one the most promising techniques for this purpose. However, wearable technologies are influenced by movement, sweat, and temperature, which would significantly affect laser accuracy in everyday use. This project aims to overcome these challenges by developing unique multi-pixel sensor arrays with wavelength extended response up to 2.5 um, a spectral range that has strong interaction with biomarkers. AI-assisted algorithms will be developed for data analysis.
[1] Emma Young, Non-invasive glucose monitoring for diabetes: five strategies under development. The Pharmaceutical Journal, 12 OCT 2017
[2] University of Birmingham, New and emerging non-invasive glucose monitoring technologies, NIHR Innovation Observatory, May 2016
Supervisor
Dr Qiandong Zhuang
Description
This project aims to establish new research in III-V/silicon integration for fully functional integrated circuits.
Realisation of efficient laser integrated with electronic circuit is the major development direction of photonic integrated circuit (PIC). It will enable fully functional PIC which has many important applications such as optical communication, on-chip sensors, imaging system, nonlinear optical switching etc. However, room-temperature high-performance lasers on Si are inferior. We will seek the feasibility of two promising technologies towards high-performance laser sources on silicon platform. One is transitional photonic lasers (either edge emitting lasers or photonic crystal surface emitting lasers) on patterned silicon wafer, and the other is surface plasmon polariton lasers on silicon wafers. The patterned epitaxial growth has demonstrated promising future of high-quality semiconductor materials on silicon, together with uniquely designed device integration, this project will demonstrate PICs with monolithically integrated lasers. Plasmon polaritons [Phys. Rev. Lett. 90, 027402, 2003] are coupled excitations consisting of charge density wave and electromagnetic field at the metal dielectric interface, which can break optical mode volume to apply optoelectronic integration [9]. Despite room temperature lasers have been demonstrated [Phys. Rev. B 85, 041301, 2012], there are several challenges hamper the realisation of high-performance plasmonic lasers on Si with low consumption, intrinsic loss of metals and heat management of the devices.
The student will gain experience and expertise in molecular beam epitaxy, semiconductor materials characterization and device fabrication in cleanroom, and photodetector assessment, together with theoretical simulation on a variety of new semiconductor photonic devices.
Supervisor
Dr Qiandong Zhuang
Description
This project aims to establish new research in wavelength-extended avalanche photodiode (APD) for single-photon counting with targeting application in biochemical sensors.
The big advance of single-photon counting is the ability to faithfully capture the single quantum of light. This technique has attracted increasing attention globally owing to the critical capability for a wide range of important applications ranging from new low-light sensing to emerging photonic quantum technologies. Its potential has been proved by several demonstrations since 2020, for instance, quantum secured internet communication over 22 Km has been established[1], long-distance single-photon imaging over 200 km has been demonstrated with high sensitivity and temporal resolution[2]; and prototype of single-photon LiDAR imaging for greenhouse gas methane mapping has been demonstrated[3]. However, the detection limit of 1650nm from well-developed Si and InGaAs ADPs restricts the deployment of potential of single photon counting. For example, biochemical sensing applications require photons at longer wavelength, e.g. mid infrared (Mid-IR), e.g. 2 – 5 um. We propose to utilize advanced type II superlattice to extend the ADP responding wavelength into MIR spectral range.
The student will gain experience and expertise in molecular beam epitaxy, semiconductor materials characterization and device fabrication in cleanroom, and photodetector assessment.
[1] Entanglement of two quantum memories via fibers over dozens of km, Yong Yu et al, Nature 578, 240 (2020)
[2] Single-photon imaging over 200 km, Zheng-ping Li et al, Optica 8, 344 (2021)
[3] Single-photon LiDAR gas imagers for continuous monitoring of industrial methane emissions, Murray K. Reed et al, Proceedings 11579, Quantum Photonics: Enabling Technologies; 115790C (2020)
Supervisor
Dr Samuel Jarvis
Description
Project summary – The goal of this PhD project is to develop highly ordered and structurally stable molecular devices. The growth of thermally and mechanically stable molecular nanostructures is a major challenge for retaining the quantum mechanical properties of molecules in real-world and demanding environments. This is especially important in nanoelectrical devices where heat and stress can damage the molecular structure, causing device failure. This PhD project aims to overcome this challenge by developing new methods for step-by-step (atom-by-atom) on-surface synthesis of covalently stabilised molecular wires and devices. Achieving this goal will address a major outstanding challenge in translating functional molecular polymers to technologically relevant materials.
Background – Thin-film molecular layers are exceptionally important for introducing high degrees of functionality to materials. Molecules can be designed with a multitude of different physical properties, ranging from high electrical conductivity, catalytic activity, tuneable optical properties, and much more [1]. These properties are determined by the electronic structure of a molecule, making them well suited for applications in quantum technologies. In particular, on-surface polymerization restricted to one and two dimensions has received considerable recent attention [2]. Not only does covalent cross-linking of molecules greatly increase their stability, on-surface polymerization also enables the growth of unique molecular structures often otherwise impossible to synthesize, including graphene nano ribbons used as molecular wires [3].
At present, the vast majority of molecular nanoscale synthesis is limited to catalytically active metal substrates, where the catalyst metal is required to activate the polymerisation reaction. This results in strong surface coupling causing molecular distortion, orbital broadening, and electrical short-circuits, thus detrimentally affecting molecular properties and severely restricting their application in physical devices. In order to fully realise nanoscale molecular devices, we must instead fabricate molecular wires directly on semiconducting substrates such as SiO2, where they can be directly integrated into nanoelectronic devices. To do this, we will build on recent findings [4] highlighting the potential to fabricate nanoscale molecular structures directly on surfaces using so-called atomic quantum clusters (AQCs).
Project Outline – This project will explore methods to direct the assembly and growth of functional molecules into nanoscale structures and devices. We will study how single molecules with well-defined quantum mechanical properties can be ‘linked’ together into rigid 1D molecular wires or 2D molecular networks, starting with porphyrin and graphene nanoribbon based wires. Single molecule and atomic scale properties will be studied with images of their detailed atomic and electronic structure (with resolution better than 0.1nm). The resulting molecular structures will provide an exciting playground to develop our fundamental understanding of quantum behaviour and molecular interactions at the atomic scale, and ultimately, provide new routes for developing nanoscale electronic devices such as field effect transistors (FETs) [5].
The selected student will have the opportunity to become trained in a broad range of techniques to study a variety of nanoscale materials. This will involve advanced scanning probe microscopy methods capable of imaging single atoms and characterising nanoscale electronic and chemical properties. This work will take place in world-leading facilities including Lancaster’s Quantum Technology Centre and the award winning IsoLab, providing some of the most advanced environments for characterisation in the world. You will work in a vibrant research group, whose research has been shortlisted for the Times Higher Education award for ‘STEM project of the year’ in 2019. You will also become highly trained in nanoscale material fabrication, ultra-high vacuum technology, X-ray spectroscopy, clean room usage, device testing, and use nano-fabrication tools to prepare devices for integration with embedded systems. Students are also expected to publish high impact journal publications and present their work at international meetings and conferences, and will receive opportunities and training for personal and research development.
Interested candidates should contact Dr Samuel Jarvis for further information.
[1] T. Kudernac, S. Lei, J. A. A. W. Elemans, and S. De Feyter, Chem. Soc. Rev. 38, 402 (2009).
[2] L. Grill and S. Hecht, Nature Chemistry, 12, 115 (2020).
[3] P. Ruffieux, S. Wang, B. Yang, C. Sánchez-Sánchez, J. Liu, T. Dienel, et al., Nature 531, 489 (2016).
[4] L. Forcieri, Q. Wu, A. Quadrelli, S. Hou, B. Mangham, N.R. Champness, D. Buceta, M.A. Lopez-Quintela, C.J. Lambert, S.P. Jarvis, Nature Chemistry (under review), (2022).
[5] J.P. Llinas, A. Fairbrother, G.B. Barin, W. Shi,. K. Lee, S. Wu, et al., Nature Communications, 8, 633 (2017).
Supervisor
Dr Samuel Jarvis
Project summary – The goal of this PhD project is to help realise a new generation of switchable molecular devices with the potential to fulfil societal needs for flexible energy harvesting materials, low-power neuromorphic computing, smart textiles, and self-powered patches for healthcare. The possibility of creating these exciting materials derives from a series of world firsts by the supervisory team, demonstrating that room-temperature quantum interference effects can be scaled up from single molecules into molecular layers with the potential to translate quantum interference effects into technologically relevant materials.
Background – Thin-film molecular layers are exceptionally important for introducing high degrees of functionality to materials. Molecules can be designed with a multitude of different physical properties, ranging from high electrical conductivity, catalytic activity, tuneable optical properties, and much more [1]. These properties are determined by the electronic structure of a molecule, making them well suited for applications in quantum technologies. In particular, a technique called on-surface polymerization has received considerable recent attention due to its ability to create unique and stable 1D and 2D molecular structures with an exciting range of quantum mechanical properties [2]. This project is an exciting opportunity to realise these new materials as part of a recently awarded £7m programme of research bringing together a world leading team in molecular electronics [3].
Project Outline – This project will explore methods for surface growth and characterisation of molecular thin films designed to optimise thermoelectric and memristive properties. The successful candidate will develop new methods to prepare highly ordered molecular films including the use of on-surface reactions that can be used to link together molecules with well-defined quantum mechanical properties into rigid 1D molecular wires or 2D molecular networks. Single molecule and atomic scale properties will be studied with Scanning Tunnelling Microscopy (STM) which provide images of their detailed atomic and electronic structure (with resolution better than 0.1nm). The resulting molecular structures will provide an exciting playground to develop our fundamental understanding of quantum behaviour and molecular interactions at the atomic scale, and ultimately, provide new routes for developing nanoscale molecular electronic devices.
The selected student will have the opportunity to become trained in a broad range of techniques to study a variety of nanoscale materials. This will involve advanced scanning probe microscopy methods capable of imaging single atoms and characterising nanoscale electronic and chemical properties. This work will take place in world-leading facilities including Lancaster’s Quantum Technology Centre and the award winning IsoLab, providing advanced environments for atomic scale characterisation. You will also become highly trained in nanoscale material fabrication, ultra-high vacuum technology and X-ray spectroscopy. Students are also expected to publish high impact journal publications and present their work at international meetings and conferences, and will receive opportunities and training for personal and research development.
[1] T. Kudernac, S. Lei, J. A. A. W. Elemans, and S. De Feyter, Chem. Soc. Rev. 38, 402 (2009).
[2] L. Grill and S. Hecht, Nature Chemistry, 12, 115 (2020).
Supervisor
Professor Jonathan Prance
Project description
The ability to cool materials to millikelvin temperatures has been the foundation of many breakthroughs in condensed matter physics and nanotechnology. At this frontier, quantum behaviour can be studied by making devices smaller and colder, increasing coherence across the system. The goal of this project is to apply a new technique – on-chip demagnetisation refrigeration – to reach temperatures below 1 millikelvin in a range of nanoelectronic structures. This will open a new temperature range for nanoscale physics.
As experiments are pushed into the sub-millikelvin regime, it becomes increasingly difficult to measure and define the temperature of a material or device. The thermal coupling between various sub-systems in can be extremely small; for example, the electrons in the metal wires contacting an on-chip structure can be at a different temperature to the electrons in the chip, the phonons in the chip, and the apparatus that you are using to cool it. This situation calls for a variety of thermometry techniques, each suited to measuring the temperature of a different physical system. The thermometers must also have extremely low heat dissipation and excellent isolation from the room temperature environment. This project will include the development of new and existing thermometry techniques that are suitable for sub-millikelvin temperatures.
Devices will be produced in the Lancaster Quantum Technology Centre cleanroom, and by our collaborators. Experiments will be conducted using the cutting-edge facilities of the Ultralow Temperature Physics group at Lancaster.
You are expected to have a strong interest in and preferably knowledge of:
- electrical measurements of nanoscale devices
- cryogenic techniques
- nanofabrication
- data acquisition using Python or MatLab
You can apply directly stating the title of the project and the name of the supervisor.
Supervisor
Professor Oleg Kolosov
Project description
Fully funded PhD position on Quantum phenomena and energy conversion in two‐dimensional materials and nanostructures. UK-Greece collaboration in European Research Council (ERC) Project.
The new PhD project is announced at Lancaster University in collaboration with the National Graphene Institute. The project focuses on the exploration of cutting-edge fundamental and applied science of “mixed physics” phenomena – electromechanical, electronic, thermal and thermoelectric - in the explosively expanding area of novel nanostructured two-dimensional materials (2DMs) and their heterostructures.
The recently discovered 2DMs – one atom thick van der Waals-bound perfect atomic layers such as graphene and transition metal dichalcogenides (TMD’s) - MoS2, Nb2Se3, InSe, etc, open unique possibilities for novel electronics, sensors and energy generation and storage. This class of materials offers unique and nature-leading physical properties – relativistic type electron mobility, the highest to the lowest known thermal conductivities, exceptional flexibility while recording strength in mechanical properties, etc.
The project focuses on the largely unexplored area of 2DM’s where physical phenomena of different nature meet – mechanical and electrical, thermal and electronic, mechanical and thermal, initiating beyond-state-of-the-art performing thermoelectrics, nanoscale actuators, super-efficient electronics, memories and sensors. For example, the highest known in nature thermal conductivity of graphene allows to precisely channel nanoscale heat in advanced processors, new TMD heterostructures have unique potential as advanced thermoelectric materials, and exceptional mechanical stiffness and low density of graphene and hexagonal boron nitride, coupled with low losses, allows to design in quantum nanoelectromechanical sensors with ultimate sensitivity limited only by the quantum mechanics laws.
The successful applicant will work at Lancaster University Physics Department within one of the world-leading groups in the exploration of physical properties of 2DM’s using scanning probe microscopy (SPM) where novel phenomena of geometrical thermoelectricity (GTE) in graphene and unique nanomechanics of domains in 2D materials heterostructures were discovered.
The project will target the manufacture of novel 2DM nanostructures including nanoconstrictions, heterostructures, suspended membranes and superconductor – 2DM devices using the state-of-the-art e-beam lithography equipped Lancaster Quantum Technology Centre facilities of National Graphene Institute and National Physical Laboratory. The developed nanostructures are studied using state-of-the-art SPMs combined with ultra-high frequency ultrasonic excitation, GHz range Laser Doppler vibrometry and super-sensitive optical interferometry, and microwave superconductor transport techniques, utilising world-leading European Microkelvin Platform (EMP) and ultra-low-nose IsoLab facilities housed at Lancaster Physics.
The Physics Department is holder of Athena SWAN Silver award and Institute of Physics JUNO Championship status and is strongly committed to fostering diversity within its community as a source of excellence, cultural enrichment, and social strength. We welcome those who would contribute to the further diversification of our department.
Contact
Professor Oleg Kolosov o.kolosov@lancaster.ac.uk for any additional enquiries. You can also apply directly stating the title of the project and the name of the supervisor.
Supervisor
Professor Manus Hayne
Project description
Vertical-cavity surface-emitting lasers (VCSELs) are high-speed, compact (low-cost) laser diodes used in laser printing, datacoms and other applications. Their implementation in the Apple iPhone X for facial recognition and motion sensing was soon replicated by other smartphone manufacturers, stimulating a growth in the VCSEL market from $775M in 2015 to an expected $4.7bn in 2024, a compound annual growth rate of 22% [1]. Nevertheless, many consumers and thus manufacturers, don’t like the small cut-out section at the top of the screen that is necessary for the implementation of the VCSEL arrays, preferring to place the VCSEL below the screen. However, achieving this requires VCSELs that emit beyond 1380 nm. Indeed, there are a host of telecoms-related and other applications such as LiDAR that have yet to benefit from VCSELs that emit in the telecoms range (1260 to 1625 nm).
VCSELs work by implementing the mirrors required for the laser cavity in repeated alternating layers of GaAs and AlxGa1-xAs, which have differing refractive indices, to make distributed Bragg reflectors that exploit interference effects. The use of GaAs/AlxGa1-xAs is strongly preferred as there is minimal lattice mismatch, despite the refractive index contrast, allowing ~100 layers to be grown with high quality. The problem is that the conventional method of extending the wavelength, via the introduction of In into the quantum wells in the active region, generates strain that limits the emission to wavelengths below 1000 nm.
The project will build on successful collaborative work between IQE and Lancaster developing telecoms wavelength GaSb quantum ring (QR) VCSELs [2]. The objective is to push the emission wavelength beyond 1380 nm and will involve the design, growth, processing and testing of individual VCSEL devices and VCSEL arrays.
This PhD is offered in collaboration with IQE. Funding for UK students is available on a competitive basis.
[1] ‘Vertical-cavity surface-emitting laser (VCSELs) market’, Transparency Market Research [Link].
[2] ‘Vertical-cavity surface-emitting laser’, M. Hayne and P. Hodgson US, Europe, Japan and S Korea patent [Link].
Supervisor
Professor Benjamin Robinson
Project summary: This is an experimental project, based in the Department of Physics at Lancaster University. You will study the electrical and thermoelectric properties of thin films of molecular materials assembled on electrode surfaces to help realise a new generation of molecular devices with the potential to fulfil societal needs for flexible energy harvesting materials.
Background: Green thermoelectricity - the sustainable generation of electricity from waste heat - has the potential to be a key enabling technology in the roadmap to the UK’s target of net zero greenhouse gases by 2050 and a pillar of the efforts to build a viable circular economy by contributing to emerging UK green-industries.
Waste heat generated by information and computing technologies (ICT) is expected to reach 30% of electricity consumption by 2025 and is widely recognised as being unsustainable. If thermoelectric (TE) energy harvesters could be developed, which perform well at relatively low temperatures (<150oC), then waste heat from ICT could be converted back into useful electricity. Energy harvested from the environment and sources such as the human body could also be used to power the internet of things and wearable devices, with engineered thermal management relevant to applications in healthcare, fashion and high-performance clothing.
This project will contribute to these technological challenges and the associated societal and economic benefits by helping to realise large area, thin-film materials and devices on rigid and flexible substrates, designed for TE energy harvesting and cooling. This overarching research challenge will be met, in part, by utilising quantum interference (QI), which introduces additional dynamical range by suppressing current flow at low bias and allows fine control of electrical and thermal conductance.
The project’s goals are aligned with the recently awarded, Lancaster-led, £7.1M EPSRC programme grant, Quantum engineering of energy-efficient molecular materials (QMol)
Project Outline: This project will focus on the thin film growth of novel organic/organometallic compounds by molecular self-assembly and Langmuir-Blodgett deposition and their subsequent characterisation by a range of surface science techniques including scanning probe microscopy.
The project is predominantly experimental, and you will gain interdisciplinary expertise spanning materials design, thin film fabrication, and nanoscale characterisation. You will benefit from Lancaster’s molecular thin film fabrication capabilities and a suite of state-of-the-art scanning probe microscopes to explore the physical processes of thermal and electrical transport in deposited ultra-thin structures. The compounds will be supplied by colleagues in the Departments of Chemistry at Oxford University and Imperial College, London. There will also be opportunities for you to work with colleagues from the Department of Physics at Imperial College to translate your thin films to practical device architectures.
Research Environment: You will benefit from a vibrant working environment and will be part of the QMol consortium incorporating partners across nine leading Universities and 11 industry partners. Through QMol, you will have the opportunity to develop skills in interdisciplinary working through close collaboration with colleagues studying the theory of quantum transport and device fabrication, as well as industry partners from both SMEs and multinational corporations.
You will be trained and supported in other academic skills such as the preparation of high-impact journal publications, and presenting your work at international meetings and conferences, and you will receive opportunities and training for personal and research development. In addition, you will have the opportunity to join in local and national outreach and engagement activities.
Lancaster University is a leading UK university, and the Physics Department at Lancaster University is one of the top in the UK for research. REF2021 rated 98% of our research outputs as world-leading or internationally excellent. The Department is ranked 4th in the UK for Physics in the Guardian University League Tables 2023.
The Department is committed to family-friendly and flexible working policies. We are also strongly committed to fostering diversity within our community as a source of excellence, cultural enrichment, and social strength. We hold an Athena SWAN Silver award and Institute of Physics Juno Champion status. We welcome those who would contribute to the further diversification of our department.
The Candidate: This project will ideally suit a candidate who has an interest in interdisciplinary experimental nanoscience. Knowledge of nanomaterials or experience in either quantum transport, scanning probe microscopy and/or self-assembly of organic monolayers would be advantageous but not compulsory as full training in a wide variety of techniques will be given. You will need to be highly motivated and be able to work as part of a team, ensuring that key milestones are reached. You will be expected to lead discussions and give regular research updates in person with the group leader and in wider research group meetings with the project consortium. The ability to plan your own workload and keep accurate scientific records is important.
General eligibility criteria: This is a highly interdisciplinary project operating at the interface of Physics, Chemistry and device engineering. Applicants would normally be expected to hold a minimum of a UK Honours degree at 2:1 level or equivalent in Physics, Chemistry, Materials Science or a related area.
Enquiries: Interested applicants are welcome to get in touch to learn more about the PhD project. Please contact Professor Benjamin Robinson, for more information
Supervisors
Professor Benjamin Robinson (Physics)
Project summary: This is an experimental project, based in the Department of Physics at Lancaster University. You will study the electrical switching properties of thin films of organometallic materials assembled on compatible electrode surfaces and in devices capped by 2D films of graphene to help realise a new generation of memristive switching devices with the potential to fulfil societal needs for next generation AI.
Background:
The advance of artificial intelligence (AI) represents the largest market opportunity in the history of humankind, estimated to be anywhere between USD 3.5 and 5.8 trillion. However, it also represents a grave environmental challenge. As a typical example, hundreds of millions of daily queries on ChatGPT can cost around 1 GWh each day, equivalent to the daily energy consumption for about 33,000 UK households. This trend is unsustainable, and new approaches are needed now.
The fundamental limitation of modern computing is the rate of data transfer between a processing unit and memory, known as the von Neumann bottleneck. This data transfer not only limits computational speed but is also highly energy intensive. To overcome this bottleneck there is a global demand for new technologies for brain-inspired, neuromorphic, computing within memory.
Memristors are one of the most promising technologies for achieving in-memory computation. Short for “memory resistors”, memristors are considered the fourth fundamental passive circuit element, alongside resistors, capacitors, and inductors. However, in contrast to traditional volatile memory technologies like RAM, which lose data when power is lost, memristors are a class of non-volatile memory, whose resistive state is maintained even when no external power is applied.
This project will contribute to the technological challenges of realising stable, efficient memristive elements formed of highly ordered thin films of organometallic molecules with highly tunable switching mechanisms.
The project’s goals are aligned with the recently awarded, Lancaster-led, £7.1M EPSRC programme grant, Quantum engineering of energy-efficient molecular materials (QMol)
Project Outline: This project will focus on the thin film growth of novel organic/organometallic compounds by molecular self-assembly and Langmuir-Blodgett deposition and their subsequent characterisation by a range of surface science techniques including scanning probe microscopy.
The project is predominantly experimental, and you will gain interdisciplinary expertise spanning materials design, thin film fabrication, and nanoscale characterisation. You will benefit from Lancaster’s molecular thin film fabrication capabilities and a suite of state-of-the-art scanning probe microscopes to explore the physical processes of thermal and electrical transport in deposited ultra-thin structures. The compounds will be supplied by colleagues in the Departments of Chemistry at Oxford University and Imperial College, London. There will also be opportunities for you to work with colleagues from the Department of Physics at Imperial College to translate your thin films to practical device architectures.
Research Environment: You will benefit from a vibrant working environment and will be part of the QMol consortium incorporating partners across nine leading Universities and 11 industry partners. Through QMol, you will have the opportunity to develop skills in interdisciplinary working through close collaboration with colleagues studying the theory of quantum transport and device fabrication, as well as industry partners from both SMEs and multinational corporations.
You will be trained and supported in other academic skills such as the preparation of high-impact journal publications, and presenting your work at international meetings and conferences, and you will receive opportunities and training for personal and research development. In addition, you will have the opportunity to join in local and national outreach and engagement activities.
Lancaster University is a leading UK university, and the Physics Department at Lancaster University is one of the top in the UK for research. REF2021 rated 98% of our research outputs as world-leading or internationally excellent. The Department is ranked 4th in the UK for Physics in the Guardian University League Tables 2023.
The Department is committed to family-friendly and flexible working policies. We are also strongly committed to fostering diversity within our community as a source of excellence, cultural enrichment, and social strength. We hold an Athena SWAN Silver award and Institute of Physics Juno Champion status. We welcome those who would contribute to the further diversification of our department.
The Candidate: This project will ideally suit a candidate who has an interest in interdisciplinary experimental nanoscience. Knowledge of nanomaterials or experience in either quantum transport, scanning probe microscopy and/or self-assembly of organic monolayers would be advantageous but not compulsory as full training in a wide variety of techniques will be given.
You will need to be highly motivated and be able to work as part of a team, ensuring that key milestones are reached. You will be expected to lead discussions and give regular research updates in person with the group leader and in wider research group meetings with the project consortium. The ability to plan your own workload and keep accurate scientific records is important.
General eligibility criteria: This is a highly interdisciplinary project operating at the interface of Physics, Chemistry and device engineering. Applicants would normally be expected to hold a minimum of a UK Honours degree at 2:1 level or equivalent in Physics, Chemistry, Materials Science or a related area.
Enquiries: Interested applicants are welcome to get in touch to learn more about the PhD project. Please contact Professor Benjamin Robinson, for more information.
Supervisor
Professor Oleg Kolosov
Project details
The new PhD project in fundamental and applied physics is announced at Lancaster University Quantum Technologie Centre (LQTC) in collaboration with the National Scientific Research Centre “Demokritos” (NCSRD) in Athens, Greece, and National Graphene Institute (NGI), UK, the birthplace of Graphene, as part of the announced collaborative European Research Council project.
The challenging and high-reward PhD project will target fundamental physics in two-dimensional (2D) materials, their nanostructures, and 2D-3D materials devices developing novel principles of advanced quantum and nanoscale energy management devices. In particular, the project will investigate largely unexplored fundamental links between electronic and phononic heat transport, electrical and nanomechanical phenomena, and thermal-electrical-mechanical energy conversion in the 2D nanostructures. The project outcomes will lead to highly efficient thermoelectric and electrocaloric devices, beyond-state-of-the-art on-chip cooling, and highly sensitive photons and phonons detectors, approaching quantum limits.
The successful applicant will work in experimental research at Lancaster University Quantum Technology Centre (LQTC) in one of the world-leading groups in the exploration of physical properties of 2DM’s using scanning probe microscopy (SPM), in direct interaction with NCSRD in Athens, the world leader in molecular beam epitaxy synthesis of 2D materials, and National Graphene Institute producing unique 2D material hetero and nanostructures. The applicant will have the opportunity to spend some time in Athens and at NGI, and collaborate with leading experimental and theoretical scientists in the field. The applicant is expected to have an excellent academic record in the Physics, Material Science or Electrical Engineering, with good experience in advanced experimentation, and good analytical skills.
The Physics Department is in the top 10 of UK Physics Departments (#4 by Guardian and Sunday Times rating and #7 by Good University Guide). It is a holder of Athena SWAN Silver award and Institute of Physics JUNO Championship status and is strongly committed to fostering diversity within its community as a source of excellence, cultural enrichment, and social strength. We welcome those who would contribute to the further diversification of our department.
Applicants are expected to have the equivalent of a first (1) or upper second (2.1) degree class in Physics or Astrophysics, supplemented by a relevant Master's-level qualification. Potential applicants are invited to apply to the physics department stating the title of the project and the name of the supervisor.
Contact Professor Oleg Kolosov for any additional enquiries
Funding note
The funding for this project is restricted to UK residents and will cover national and international secondments to the NGI and NCSRD in Athens.