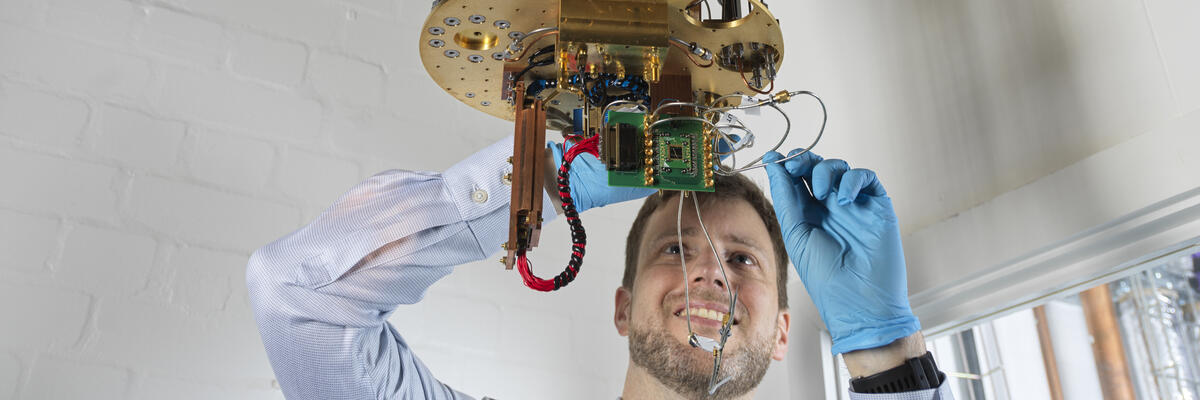
About us
We perform experiments on superfluids and other materials with applications in areas such as nanoelectronics, cosmology and turbulence.
The group has a strong international reputation for performing state-of-the-art experiments at the lowest achievable temperatures. Our custom made dilution refrigerators, built in-house, achieve world-record low temperatures. We have pioneered several innovative approaches, including:
- 'Lancaster-style' demagnetisation stages to cool superfluid helium-3 to record low temperatures
- 'heat-flush' procedures to produce highly purified helium-4
- ion transport measurement methods for quantum fluids
- novel NMR systems
- various mechanical oscillator techniques which provide extremely sensitive thermometry and bolometry at microkelvin temperatures
We are well known for providing these sub-millikelvin low temperature environments with advanced in-house cryogenic engineering, and for our accompanying expertise in ultra-sensitive measurement techniques and the development of specialised instrumentation.
Creating, controlling and exploiting the ultra-low temperature environment has proven crucial for the research and development of quantum-enhanced devices. Our platform technology provides the extreme cold and isolation necessary to probe the subtle quantum behaviours that are otherwise hidden by thermal fluctuations or external disturbance.
We have a broad research portfolio in low temperature physics and specialise in quantum fluids and solids research. We have performed ground-breaking analysis on numerous topics, including
- superfluid analogues of cosmological processes
- ion and vortex ring dynamics
- ballistic quasiparticle beams
- exotic superfluid spin phenomena
- superfluid phase nucleation
- phase boundary dynamics
- wave turbulence
- quantum turbulence
Key Research
- Cooling and sensitive measurement techniques
- Quantum fluids and solids
- Superfluid 3He
- Superfluid 4He
- Properties of materials at ultra-low temperatures
- MEMS and NEMS devices at low temperatures
- Extremely cold devices and associated quantum technologies
- Development of sensitive measurement techniques
-
Group Members
Loading People
PhD Opportunities
Accordion
-
Nanomechanical sensors for magnetic resonance microscopy
Supervisor
Professor Edward Laird
Description
Magnetic resonance imaging (MRI) is a powerful and non-invasive technique for looking inside the human body. If we could make a microscope that works on the same principle, we would be able to do something that is presently impossible – to look inside cells, viruses, and potentially even individual molecules and identify the atoms from which they are made. Unfortunately, MRI machines cannot simply be made smaller, because as their radio antennas are shrunk they become less sensitive. For this reason, the resolution of conventional MRI is still far below that of other kinds of microscope.
To develop an MRI microscope, we need to develop a new kind of device that measures the same effect with much higher resolution. Such an approach is magnetic resonance force microscopy. In this technique, a tiny nano-magnet is attached to a delicate mechanical spring and positioned as close as possible to the specimen being measured. As the nuclei in the specimen precess, their magnetic field deflects the nano-magnet, thus creating a measurable signal.
To construct a microscope based on this principle is still a formidable challenge. For each nucleus in the specimen, the force exerted on the nano-magnet is roughly one zepto-Newton. We aim to detect such a force by using the lightest, most delicate spring that can be fabricated – a single carbon nanotube. This project will develop nanotube force sensors and the associated quantum electronics to measure them. The two central physics challenges are to attach a nano-magnet to a nanotube spring and to measure its tiny deflection. To overcome them, we seek highly motivated graduates in physics or related fields with curiosity, grit, and a passion for making new discoveries through experiment.
We have a strong track record of high-profile publications by PhD students. We have access to excellent facilities for nanofabrication, electronics, and low-temperature measurement. These include:
- The state-of-the-art cleanroom of Lancaster’s Quantum Technology Centre.
- New cryogen-free dilution refrigerators optimised for high-speed quantum electronics and equipped with ultra-sensitive superconducting amplifiers.
- Extensive collaborations with low-temperature and quantum physicists in Lancaster and beyond.
Within this project, you will work in the Low Temperature Physics and Quantum Nanotechnology groups at Lancaster. You will receive a thorough training, supported by state-of-the art equipment, in quantum electronics, low-temperature physics, nanofabrication, and scientific communication. Through your research in this project, you will have the opportunity to contribute to a physics-based technology with profound potential in materials science and biology.
Further information: The quantum electronic sensors group
-
Probing quantum fluids with vibrating carbon nanotubes
Supervisor
Professor Edward Laird
Description
Superfluidity is among the most fascinating manifestations of collective quantum behaviour. Many behaviours that appear fundamental to our universe, such as gauge invariance and the Higgs mechanism, have emergent analogues in the superfluid. Other superfluid features mimic important properties of condensed matter, such as topological defects; others may even simulate the interiors of neutron stars, or the early universe.
Like all quantum systems, a superfluid is fundamentally characterised by its excitations, or quasiparticles. A powerful way to study these quasiparticles is to immerse a vibrating wire inside the superfluid; its mechanical damping reveals the amount of energy deposited in the superfluid, and therefore tells us about the quasiparticles that have been created.
We are developing tools to measure superfluids on the mesoscopic scale, i.e. between the size of atoms and the scale of the superfluid coherence length. Our plan is to use the smallest vibrating wires that can be created, namely vibrating carbon nanotubes. Because they are so small, they can respond to tiny damping forces. We can also measure them using quantum electronic circuits, which lets us detect their motion with high sensitivity.
In this project, we will first study the bosonic superfluid 4He, whose quasiparticles are phonons and rotons. We will then study the fermionic superfluid 3He, whose quasiparticles are more exotic. Our aim is to learn how we can create different kinds of quasiparticles by changing the size and vibration frequency of our nanotube.
We have a strong track record of high-profile publications by PhD students. We have access to excellent facilities for nanofabrication, electronics, and low-temperature measurement. You will receive a thorough training, supported by state-of-the art equipment, in quantum electronics, low-temperature physics, nanofabrication, and scientific communication. Our facilities include:
- The state-of-the-art cleanroom of Lancaster’s Quantum Technology Centre.
- New cryogen-free dilution refrigerators optimised for high-speed quantum electronics and equipped with ultra-sensitive superconducting amplifiers and superfluid sample cells.
- Nuclear demagnetisation refrigerators that can access some of the coldest temperatures in the universe.
- Extensive collaborations with low-temperature and quantum physicists in Lancaster and beyond.
Through your research in this project, you will have the opportunity to contribute to a physics-based technology with profound potential in materials science and biology.
Further information: The quantum electronic sensors group
-
A miniature atomic clock using endohedral fullerene molecules
Supervisor
Professor Edward Laird
Description
Atomic clocks are the most precise scientific instruments ever made, and are key to advanced technologies for navigation, communication, and radar. The most accurate atomic clocks cost millions of pounds and take up entire rooms, but an important goal for this research field is to develop miniature, portable clocks. This is a major challenge for quantum science and technology.
This PhD project will pursue a new approach to create a clock that will fit on a chip. Present-day atomic clocks are based on atomic vapours confined in a vacuum chamber. Our new approach is to use electron and nuclear spins in endohedral fullerene molecules – nature’s atom traps – whose energy levels offer an exquisitely stable frequency reference. To make this novel approach work, we must overcome a range of physics and engineering challenges, including detecting spin resonance from a small number of spins, identifying the energy levels involved, and miniaturizing the control electronics and magnet. The reward will be a completely new technology with a wide range of civilian and military uses. We are looking for a candidate who has a strong interest in applying quantum physics in new technology and is motivated to develop the new and demanding electronic measurement techniques that will be necessary.
Further information:
-
Building cryogenic electronics using 2D materials
Supervisor
Dr Michael Thompson
Description
There is a growing demand for electronic components that operate are cryogenic temperatures, from analogue amplifiers to digital control circuits for quantum computing. Existing electronic components are manufactured using semiconductors, mostly silicon, that either don’t work at all, or work poorly at very low temperatures. Two-dimensional materials, such as graphene, have been used for building transistors and even more complex components, with comparable performance to existing semiconductors. However, unlike existing semiconductor components, these materials continue to function as well, if not better, at very low temperatures. The aim of this project is to build cryogenic electronics using these 2D materials, in particular, using commercially available wafer-scale graphene to build analogue amplifiers.
This project will make use of Lancaster’s cleanroom for fabrication and the IsoLab facility for device characterisation. IsoLab is equipped with a dilution refrigerator capable of cooling devices down to 10 mK and is housed inside an electromagnetically shielded room. The filtered mains circuits and dedicated ground nest make this facility the ideal location for testing low-noise cryogenic electronics.
For this project, a student will learn to fabricate nanoelectronic devices using both 2D materials and semiconductors and characterisation of these inside a cryogenic refrigerator. This work is closely linked to existing collaborations with the National Graphene Institute in Manchester and the European Microkelvin Platform project and the student will have the opportunity to engage with these collaborations.
You are expected to have a strong interest and preferably knowledge in:
electrical measurements of nanoscale devices
cryogenic techniques
nanofabrication
data acquisition using Python or similar
-
Ultralow temperatures in nanoelectronic devices
Supervisor
Dr Jonathan Prance
Description
The ability to cool materials to millikelvin temperatures has been the foundation of many breakthroughs in condensed matter physics and nanotechnology. At this frontier, quantum behaviour can be studied by making devices smaller and colder, increasing coherence across the system. The goal of this project is to apply a new technique – on-chip demagnetisation refrigeration – to reach temperatures below 1 millikelvin in a range of nanoelectronic structures. This will open a new temperature range for nanoscale physics.
As experiments are pushed into the sub-millikelvin regime, it becomes increasingly difficult to measure and define the temperature of a material or device. The thermal coupling between various sub-systems in can be extremely small; for example, the electrons in the metal wires contacting an on-chip structure can be at a different temperature to the electrons in the chip, the phonons in the chip, and the apparatus that you are using to cool it. This situation calls for a variety of thermometry techniques, each suited to measuring the temperature of a different physical system. The thermometers must also have extremely low heat dissipation and excellent isolation from the room temperature environment. This project will include the development of new and existing thermometry techniques that are suitable for sub-millikelvin temperatures.
Devices will be produced in the Lancaster Quantum Technology Centre cleanroom, and by our collaborators. Experiments will be conducted using the cutting-edge facilities of the Ultralow Temperature Physics group at Lancaster.
You are expected to have a strong interest in and preferably knowledge of:
- electrical measurements of nanoscale devices
- cryogenic techniques
- nanofabrication
- data acquisition using Python or MatLab
-
Flight in superfluids
Supervisor
Dr Dmitry Zmeev
Description
The project is to create and perform experiments with new types of superconducting probes for quantum liquids: precisely controllable levitators working at sub-millikelvin temperatures. Recently we have made a significant progress in the development of these instruments and we will explore several outstanding problems.
Firstly, pinning and nucleation of quantum vortices in superfluid helium-4. Currently, there are two contradictory pictures of how quantum vortices attach (‘pin’) to surfaces and how it affects their motion. A levitating sphere offers a new type of experimental topology and has the power to resolve this issue.
Secondly, the question of existence of a lift force in a superfluid remains open. We will build a hydrofoil, move it through superfluid and observe whether the lift exists.
Thirdly, the surface-bound states in superfluid helium-3, the coldest liquid in the Universe, at microkelvin temperatures represent a largely unexplored physical system with potentially extremely unusual properties. We have recently demonstrated how to probe this system by driving it out of equilibrium, and the new instruments promise to enhance our capabilities.
-
Scalable superconducting electronics with graphene Josephson junctions
Supervisor
Dr Michael Thompson
Description
Josephson junctions are a key component in superconducting electronics and are used in superconducting qubits, superconducting quantum interference devices, Josephson parametric amplifiers and many more. Junctions formed with graphene can have their critical current tuned using a local gate, creating junctions whose properties can be varied during operation. This has the potential to enhance existing technologies or open up possibilities for creating entirely new devices.
While such junctions have already been demonstrated, these use exfoliated graphene flakes, which is not a scalable technology and makes implementation of these junctions impractical for applications outside of fundamental research. Graphene is available in large areas, grown by chemical vapour deposition and while the quality is not as high as exfoliated flakes, it is possible to make junctions using this material. This opens up the opportunity for building superconducting electronics with tunable junctions at scale. This project aims to develop a process for fabricating graphene-superconductor junctions with low resistance contacts using CVD graphene and once established, design and build electronics devices.
This project will make use of Lancaster’s cleanroom for fabrication and the IsoLab facility for device characterisation. IsoLab is equipped with a dilution refrigerator capable of cooling devices down to 10 mK and is housed inside an electromagnetically shielded room. The filtered mains circuits and dedicated ground nest make this facility the ideal location for testing low-noise cryogenic electronics. For this project, a student will learn to fabricate nanoelectronic devices using 2D materials and characterisation of these inside a cryogenic refrigerator.
You are expected to have a strong interest in:
- electrical measurements of nanoscale devices
- cryogenic techniques
- nanofabrication
- data acquisition using Python or similar
-
Cosmology in superfluid 3He
Supervisor
Dr Samuli Autti
Description
In this PhD project, the student will work as a member of the QUEST-DMC team in Lancaster, based in the ULT laboratory. The project entails constructing and using magnetic confinement to study superfluid 3He at ultra-low temperatures. Superfluid 3He is perhaps the most versatile macroscopic quantum system in the laboratory, and this project will have direct consequences for seemingly distant fields such as particle physics and cosmology. The aim is to show that a first order phase transition can take place in the absence of external influence and that this process is described by the elusive homogeneous nucleation theory. This would redefine how we understand phase transitions in pure systems such as the Early Universe, where such a phase transition possibly left behind gravitational waves that a specialist satellite mission may still be able to observe. The work done will simultaneously technologically contribute towards using the superfluid as a dark matter detector in a large inter-university project in the UK.
Postgraduate Training
We run training sessions for postgraduate students throughout the year, covering both specific low temperature technology and more general research skills.
You can also attend a series of lectures jointly organised with the University of Manchester. These will allow you to meet students from other institutions, as well as being taught new material that may be of use to you in future years.
The nature of the training is dependent on your project requirements and the skills you will need to make progress in your work. You will engage in a continual discussion with us to ensure that we provide the most relevant and practical training. The Faculty of Science and Technology, ISS, and the Library offer additional training.
You will receive training in a wide range of formats on topics of interest to your project:
- Physics of superfluids and superconductors
- Essential cryogenic safety and safe handling of cryogenic liquids
- Operation of sophisticated low temperature refrigeration equipment
- Fabrication of NEMs and MEMs devices
- Experimental instrumentation design and construction
- Innovative measurement techniques
- Data acquisition techniques
- Data analysis and computer-based simulation techniques
- Preparation of manuscripts, posters and oral presentations
You could attend a variety of scientific conferences, allowing you to present your work. If necessary, you will receive additional support and advice on the preparation of posters and oral presentations. You also have the chance to develop their presentation skills via participation in the Department’s public understanding of physics outreach programme.
New tutorials, workshops and conferences include:
- Annual Institute of Physics Low Temperature Techniques Course
- Annual European School and Workshop in Cryogenics and Quantum Engineering
- EuHIT School on Turbulence provides an opportunity for high-quality lectures on experimental techniques, numerical methods in fluid dynamics and the theory of turbulence
- Triennial International Conference on Low Temperature Physics
- Satellite LT28 on Ultralow Temperature Physics: ULT 2025 Frontiers of Low Temperature Physics
- 2024 International Symposium on Quantum Fluids and Solids